First observations of the spontaneous discharge of an electrometer
The torsion balance electrometer Coulomb used to…
Know moreThe source: Earth, atmosphere or outer space?
In studying electrical conduction through air in…
Know moreTheodor Wulf, a new electrometer, and the Eiffel tower
The original Wulf electroscope (Image: Wikimedia…
Know moreCharles Thomson Rees Wilson sees particle tracks
Cloud formed on ions due to α-Rays (Image: CTR…
Know moreRobert Millikan coins the term ‘cosmic rays’
Robert Millikan originally set about to disprove…
Know moreGeiger-Müller counters and the coincidence technique
Bothe and Kolhorster’s experiment (Image: L.…
Know moreBruno Rossi: Cosmic rays are positive charged particles
Bruno Rossi (Image: Wikimedia Commons) Rossi’s…
Know moreCarl Anderson and Seth Neddermeyer discover the muon
The muon was discovered as a constituent of…
Know morePierre Auger and colleagues demonstrate extensive air showers
Pierre Auger, who had positioned particle…
Know moreClifford Butler and George Rochester discover the kaon, first strange particle
Stereoscopic photographs showing an unusual fork…
Know moreAir Cherenkov discovery Galbraith & Jelley
The detector used for the first observations of…
Know moreJohn Linsley detects the first 10^20 eV cosmic ray
John Linsley detected first cosmic ray with an…
Know moreDetection of high-energy gamma rays from Crab Nebula
Astrophysicists detected pulsed gamma-ray…
Know moreFly’s Eye detects record-breaking cosmic ray: 3.2 x 10^20 eV
The Fly's Eye Mirrors (Image: Courtesy of…
Know moreBaikal – first underwater neutrino telescope
Satellite image showing spring ice melt underway…
Know morePierre Auger Observatory completes its 100th detector
Upon completing its 100th surface detector, the…
Know moreThe Cosmics Leaving Outdoor Droplets (CLOUD) experiment as shown by Jasper Kirkby (spokesperson). (Image: CERN) New experiment to investigate the effect of galactic cosmic rays on clouds and climate
The CLOUD (Cosmics Leaving OUtdoor Droplets)…
Know morePierre Auger Observatory discovers extragalactic origin of highest-energy cosmic rays
In November 2007, the Auger project published…
Know moreLaunch of the Alphamagnetic Spectrometer (AMS)
AMS during tests at the University of Geneva,…
Know moreIceCube measures 5σ significance level for the observation of extraterrestrial neutrinos
Inspired by the 1PeV events, IceCube began a…
Know moreAMS experiment unveils new territories in the flux of cosmic rays
The Alpha Magnetic Spectrometer (AMS)…
Know moreIceCube reports first evidence of a source of high-energy cosmic neutrinos
Scientists at IceCube observatory and…
Know more
Embed this timeline
First observations of the spontaneous discharge of an electrometer
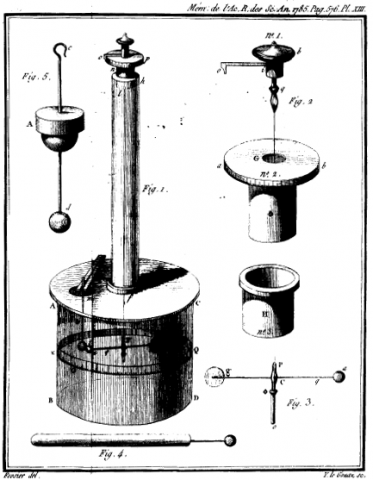
Becquerel discovers radioactivity
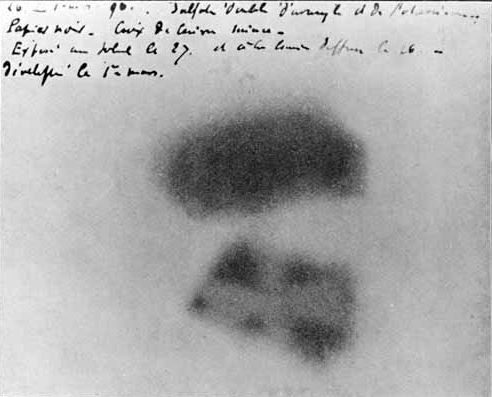
The source: Earth, atmosphere or outer space?
Theodor Wulf, a new electrometer, and the Eiffel tower
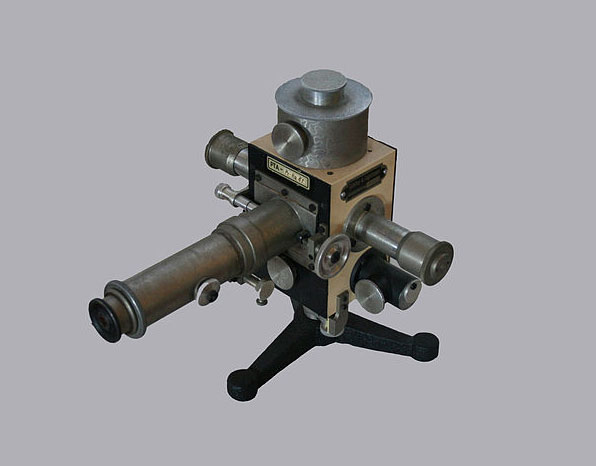
Albert Gockel’s flights
Pacini and underwater measurements
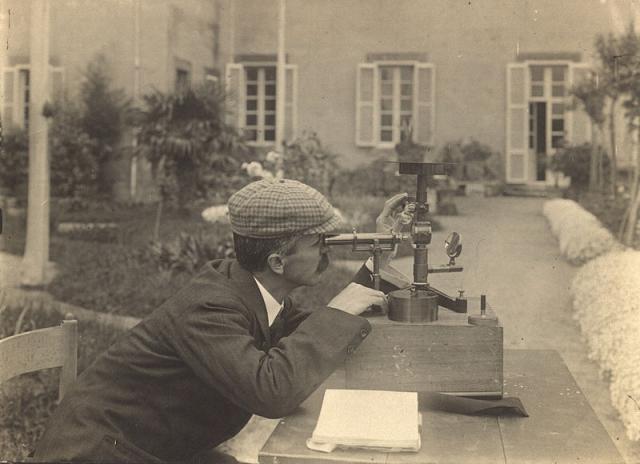
Charles Thomson Rees Wilson sees particle tracks
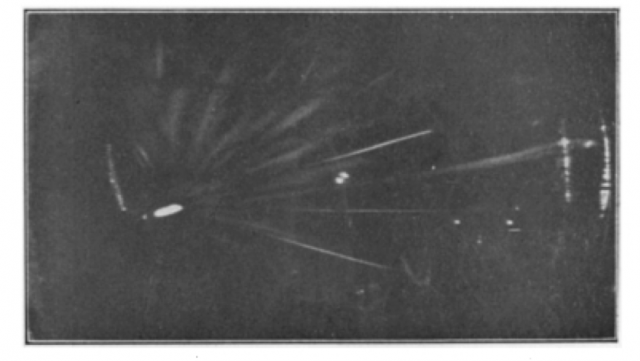
Victor Hess discovers cosmic rays
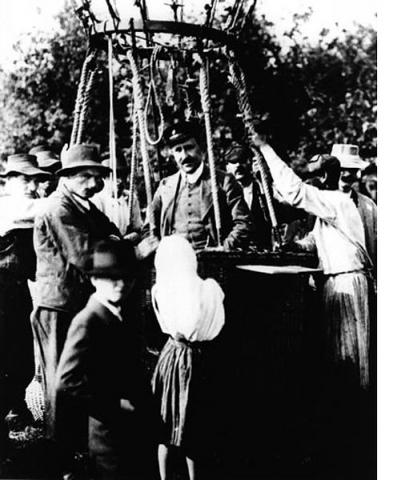
Kolhörster confirms Hess’s findings
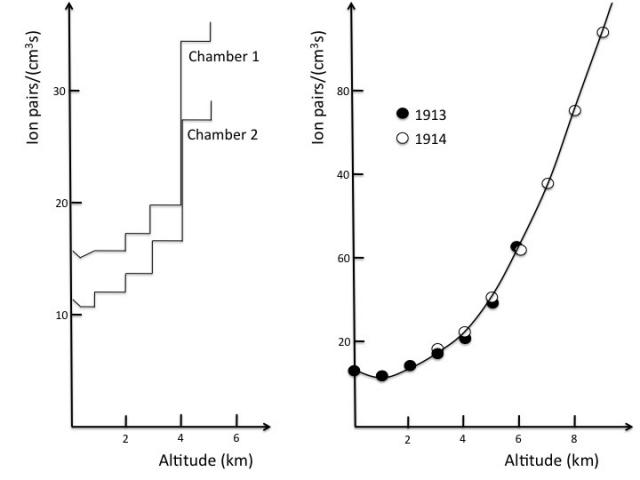
Robert Millikan coins the term ‘cosmic rays’
Geiger-Müller counters and the coincidence technique
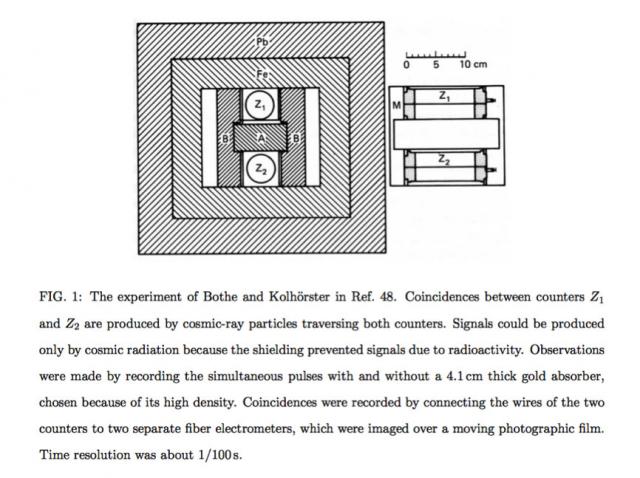
Carl Anderson discovers the positron
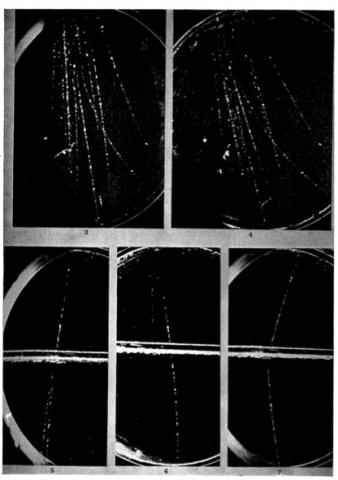
Bruno Rossi: Cosmic rays are positive charged particles
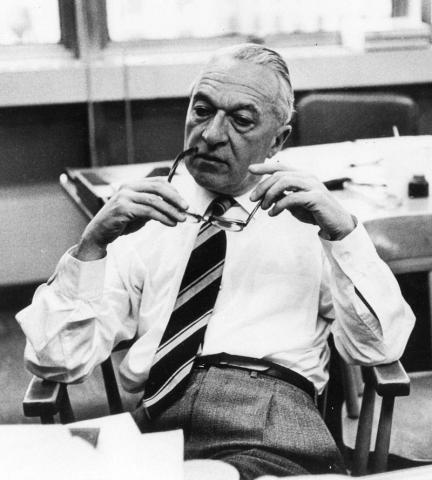
Carl Anderson and Seth Neddermeyer discover the muon
Pierre Auger and colleagues demonstrate extensive air showers
Clifford Butler and George Rochester discover the kaon, first strange particle
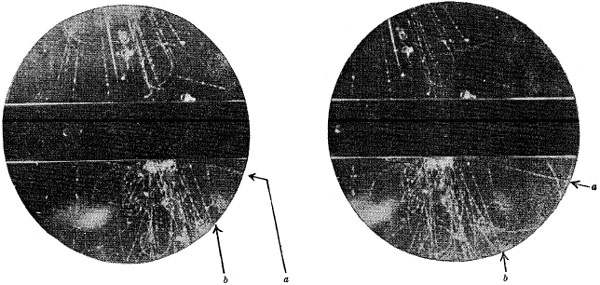
Air Cherenkov discovery Galbraith & Jelley
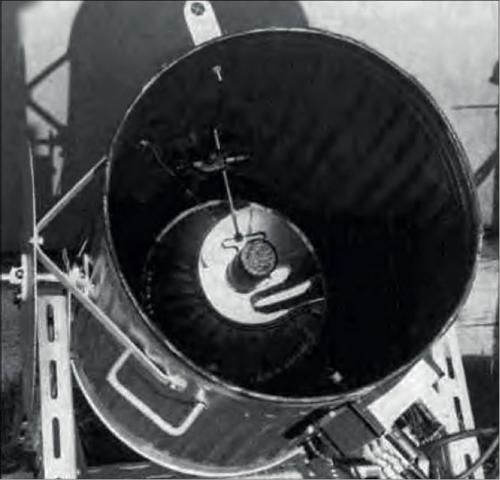
John Linsley detects the first 10^20 eV cosmic ray
Detection of high-energy gamma rays from Crab Nebula
Fly’s Eye detects record-breaking cosmic ray: 3.2 x 10^20 eV
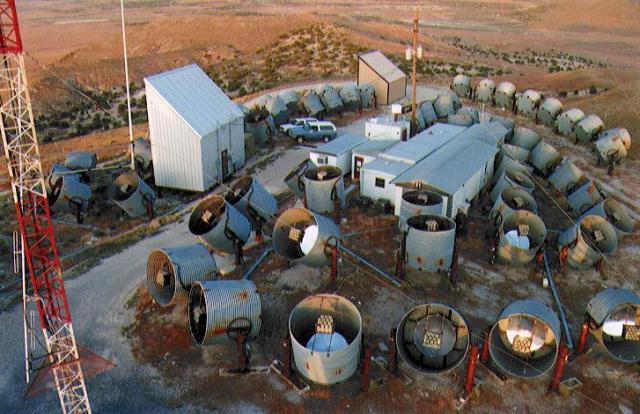
The AGASA Cosmic-Ray event
Baikal – first underwater neutrino telescope
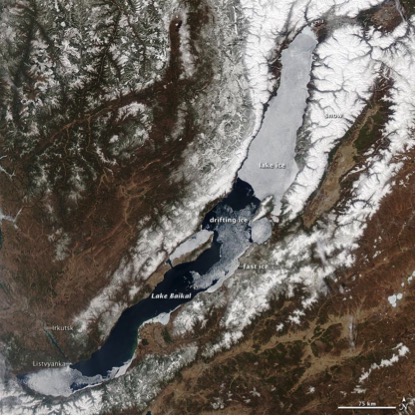
AMANDA sees first neutrinos in ice
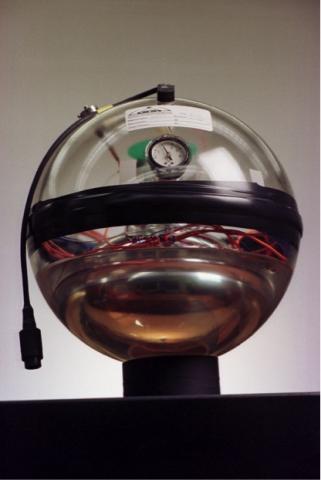
Pierre Auger Observatory completes its 100th detector
New experiment to investigate the effect of galactic cosmic rays on clouds and climate
Pierre Auger Observatory discovers extragalactic origin of highest-energy cosmic rays
IceCube construction completed
Launch of the Alphamagnetic Spectrometer (AMS)
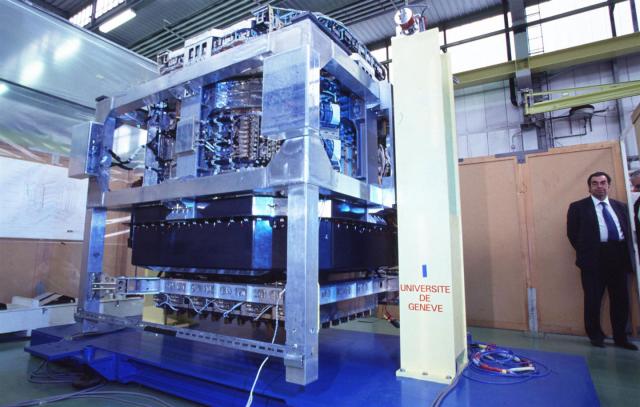